Geological transformation, ice ages and how they reveal past climates, and the rich diversity of Ireland’s geology with Professor Frank McDermott, School of Earth Sciences
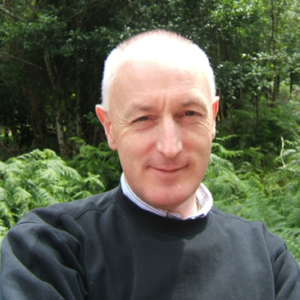
Q. How did you get interested in your area of earth sciences?
A. I’ve always been curious about rocks and minerals which got me interested in studying geology and earth sciences. I had a box of minerals under my bed when I was a child, that kind of thing. Later I started collecting shiny minerals and found out later that they were sulphide ore minerals that contained metals. I didn’t study geography in secondary school. There would have been a small amount of geology in the Leaving Cert geography course at the time, but I didn’t do that at second level. I did physics and chemistry and was very interested in both. I didn’t do biology because the choice was physics or biology.
I think my curiosity about the materials around me ultimately led me to geology at third level and then to geochemistry, which is the link between geology and chemistry. Some knowledge of chemistry is good for mineralogy as well, because it helps you understand what’s in minerals and how they might form in nature.
Ultimately, I did a PhD at the Open University (OU) in the UK, on igneous petrology with a focus on granites in the Damara belt of Namibia. There was an economic geology aspect to it because it turns out there is important uranium mineralisation in some of the granites in Namibia in southwest Africa. At that stage I was thinking of economic geology as a career, but I’d already worked in mineral exploration for a couple of years before my PhD and as time went on, I got more into academic research and I did a post-doc after my PhD, also in the OU.
During my first post-doc at the OU, I improved a laboratory method for uranium-thorium dating by mass-spectrometry. Traditionally this work would have been done by alpha-particle counting methods that were slow and not very precise. The uranium-thorium method allows you to date recent sediments as well as recent igneous rocks; by recent I mean less than about half a million years old! That work got me into the more recent part of the geological record. Dating samples like corals for example to look at relative sea-level change and deposits such as stalagmites in caves, that sort of thing. The aim was to first work out their depositional age and then trying to figure out what they can tell us about past environmental and climatic conditions using a variety of geochemical measurements.
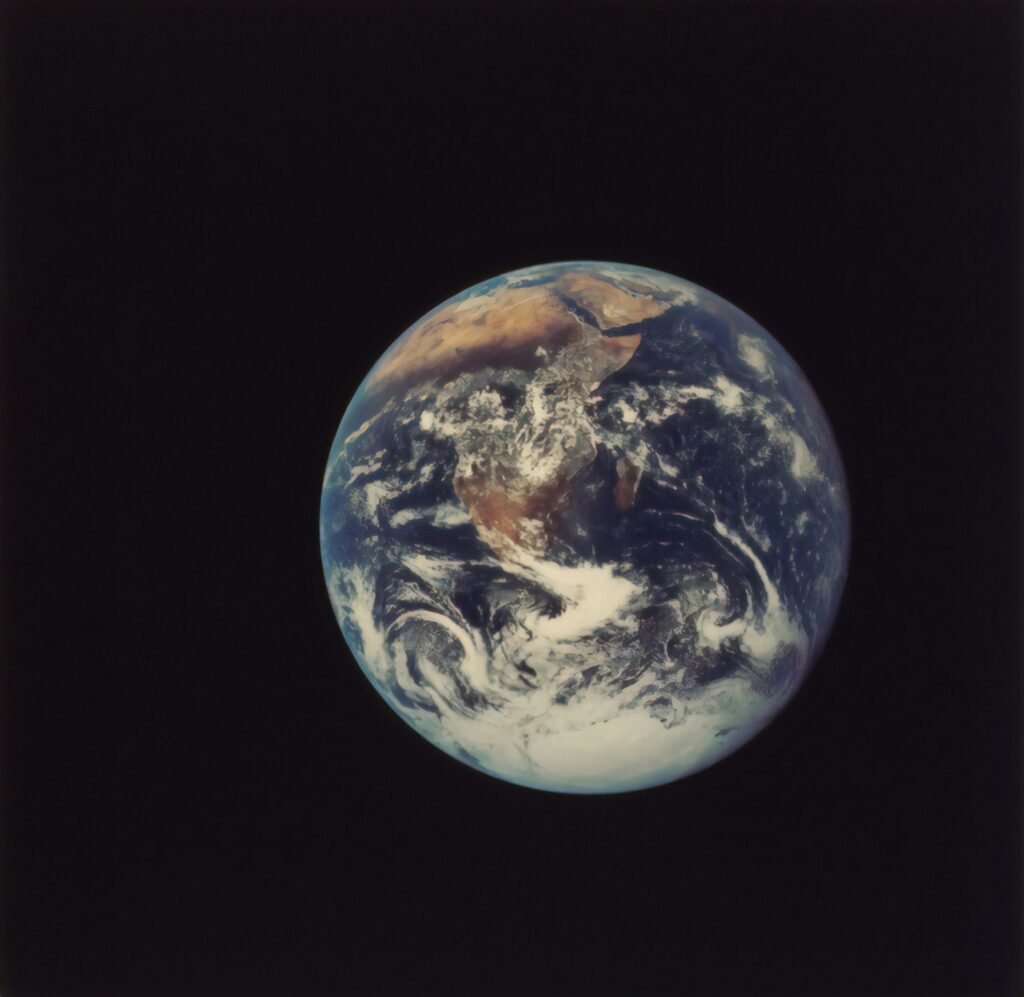
“[With isotopes].. you can trace how different chemical elements move around the system, between the solid Earth, the hydrosphere, the atmosphere etc, moving around and exchanging between different parts of the Earth on different timescales.”
Q. Does this all broadly fit into geochemistry?
A. Yes, all of that work used geochemistry as a dating tool. In geology, we can use isotopes in two ways. We use them as chronometers to estimate of the age of a mineral or rock. That’s how we know the age of the Earth for example; by looking at decay of certain isotopes of uranium to isotopes of lead in minerals like zircons. That’s just one application of geochemistry.
The other use of isotopes in geology would be as tracers. In that way you can trace different metals or different chemical elements around the earth system depending on their isotopic ‘flavour’, as it were. That works for all sorts of atoms, from light elements like carbon and oxygen all the way to heavy elements like uranium. You can use stable isotope ratios or ratios of isotopes that involve radioactive decay. You can trace how different chemical elements move around the system, between the solid Earth, the hydrosphere, the atmosphere and so on, moving around and exchanging between different parts of the Earth on different timescales.
As an example, if you look at a chemical element like strontium which is chemically similar to calcium, and a relatively abundant trace element in many geological materials. It tends to have a certain isotopic ratio or isotopic flavour if it comes from the Earth’s mantle for example, whereas if it comes from the Earth’s continental crust it will have a different isotopic flavour that reflects how parent/daughter element ratios get changed or fractionated when rocks melt as well as the accumulated effect of radioactive decay and radiogenic production over time. You can trace the movement of materials around the Earth using Sr and other isotope systems which is incredibly useful for all sorts of applications in the Earth Sciences. We have a dedicated laboratory, the National Centre for Isotope Geochemistry at UCD, where we do this kind of specialised work.
Q. How does this dating work? Does it tell when a material is formed into its current state?
A. That’s right. Normally we are dating of the time of crystallisation or deposition of a mineral. If we date, let’s say, a mineral such as a tiny zircon crystal in a granite we get information about when the mineral as a result of solidification from the granite magma – in essence we’ve then dated the age of the granite. On the other hand, in a near-surface sedimentary system the event dated might be a biogeochemical event, for example when an organism’s carbonate shell or bio-mineralised fossil hard-part, was precipitated from seawater.
Effectively we’re nailing down the time when the parent and daughter chemical elements that drive the isotopic system of interest became fixed and stopped moving around; when the system of interest becomes closed. The isotopic clock starts effectively at that time. We also have methods to correct for the presence of any initial radiogenic isotopes that could have been present in the system at the start, but we try to minimise this correction by choosing our minerals carefully.
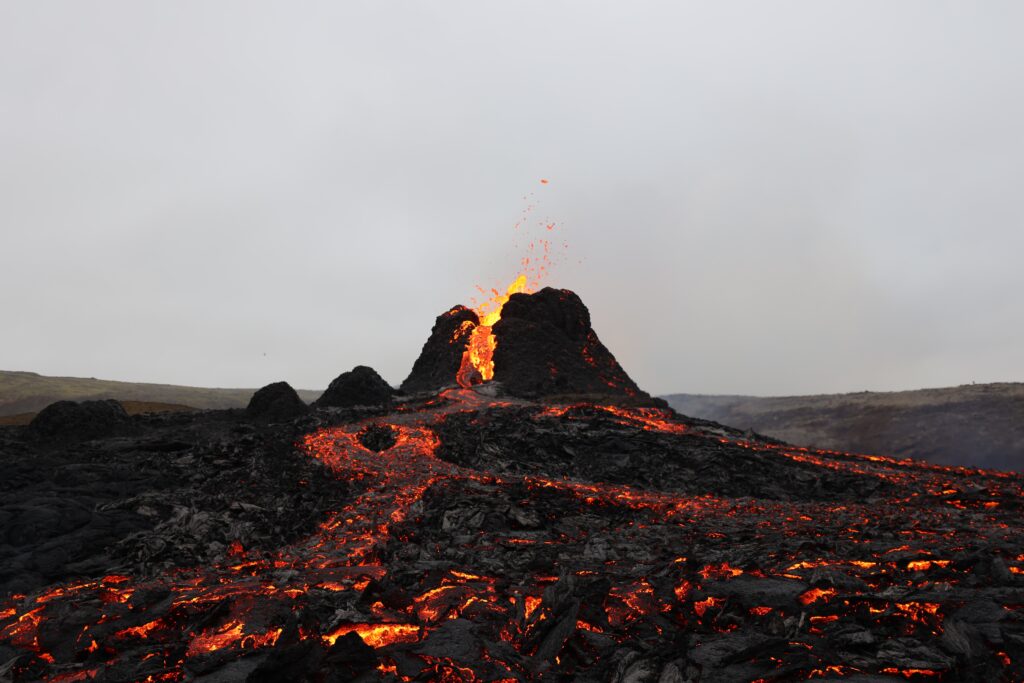
“The early Earth differentiated into the iron and nickel-rich metallic core and outer silicate-rich layers. The lighter elements like aluminium and silicon migrate outwards towards the crust, which is why the Earth’s core is much denser than the crust.”
Q. If you could go back to the beginning, what is everything made of? Asking as a non-geologist!
A. Ultimately it all comes from the big bang some 13.7 billion years ago. Earth’s story started much later of course, a mere 4.6 billion years ago! Materials were hoovered up as the planets in the Solar System formed and chemical differentiation of the elements happened over time. The early Earth differentiated into the iron and nickel-rich metallic core and outer silicate-rich layers. The lighter elements like aluminium and silicon migrate outwards towards the crust, which is why the Earth’s core is much denser than the crust.
Some elements have radioactive atoms or isotopes that decay over time – take an example of uranium and lead. Let’s imagine our zircon crystal again, a tiny crystal that’s perhaps 100 microns or so in size, and it formed when a granitic magma solidified, let’s say, back in the Archaean, before 2.5 billion years ago. When that zircon crystal formed, it incorporated small amounts of uranium because uranium can fit inside its crystal structure, but the daughter isotopes of lead were more or less excluded. It starts off with a high uranium to lead ratio. Over time some of that uranium decays to isotopes of lead, so my measuring the lead 206 to 204 or lead 207 to 204 in our mass-spectrometers we can calculate the age of zircon crystallisation.
The beauty of this system is that it has an in-built check – we have two independent chronometers arising from the radioactive decay of two isotopes of uranium 238 and 235. If the system remains closed and everything works as it should, both chronometers should give the same age and that greatly improves our confidence in these ages.
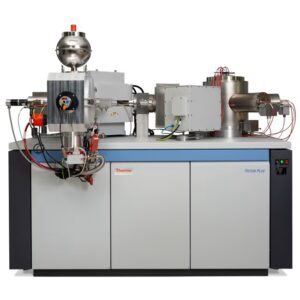
“We get to study processes at a vast range of scales, from tiny nano-meter scales up to the size of continents; thousands of kilometers.”
Q. Do you do a lot of experimental work?
A. Yes. The nice thing about geology as a subject is that we get to study processes at a vast range of scales, from tiny nano-meter scales up to the size of continents; thousands of kilometers. Experimental work encompasses everything from going out in the field with a hammer to take large samples to the painstaking and meticulous low-blank chemistry in a clean lab or using a laser to ablate and analyse micron-scale domains in crystals. The lab work often involves careful chemical separations followed by analysis of minute amounts of separated elements using mass-spectrometers and other laboratory instruments. There’s a huge range of activities on all scales imaginable – never a dull moment!
“Geological time is vast; it’s hard for humans to imagine a million years of time, but the Earth is 4.6 billion years old! […] There’s an international commission on stratigraphy which decides on where to place the boundaries are between the different epochs, different geological epoch using type sections.”
A. Geology breaks the history of the earth into time periods, eons, eras and epochs etc. Is there general consensus on these time periods and also how are they differentiated?
A. Geological time is vast; it’s hard for humans to imagine a million years of time, but the Earth is 4.6 billion years old! There is consensus for the most part about the geological epochs and the remaining arguments are mainly about the details. There’s an international commission on stratigraphy which decides on where to place the boundaries are between the different epochs, different geological epoch using type sections. They’re often based on a succession of rocks; say in China or Europe or in the US. These rocks are known from their fossils to be of that age, approximately, and then they try to define a good type-section or a place where you can really pin down the change from one geological epoch to the next.
That’s often marked by a change in the character of the sediments. So sedimentary rocks might change their colour, or their texture, or sometimes there’s a substantial time gap between them, indicating than an event of some kind happened. It could be climate related or an abrupt change in depositional conditions, or there might have been extreme events like vast volcanic outpourings for example, or even a meteorite impact event, which would reset everything. The radiogenic isotope systems that I mentioned previously help us to put hard numbers, in millions of years on the key geological boundaries.
Q. What about Anthropocene as a term, is it accepted widely?
A. The Anthropocene is somewhat controversial in that regard. It hasn’t been formally accepted as an epoch in Geology. The term is used to refer to the time in which humans have had a big impact on Earth systems. The problem is that it’s difficult to pin down the record within the geological strata. If it’s not there in the rocks, or if we can’t point to a place where everybody agrees that this is where a big change occurred, should we call it a new epoch? It’s still a useful term though because it conveys the realisation that humans have had and are continuing to exert a big impact on Earth systems, but currently it is not a formal geological epoch.
“[The Anthropocene] conveys the realisation that humans have had and are continuing to exert a big impact on Earth systems, but currently it is not a formal geological epoch. […] Regardless, there is agreement that humans are having a huge impact, especially on the faster moving parts of the Earth system, the atmosphere, and the oceans.”
Q. When do people think the line is?
A. Some people say since the industrial revolution in the 19th century. Others would say we should go further back in time to when we started to have widespread deforestation for agriculture, thousands of years ago, after the last ice age when the first farmers modified the landscape at scale, maybe that’s when we should have it. Others would say we should have it as recently as when we first began using and dumping plastics in sediments, or when we had the bomb spike; the atomic bomb testing of the early 1960s that cause a big spike in atmospheric radiocarbon content. So far there’s no general agreement on where it should be.
Regardless, there is agreement that humans are having a huge impact, especially on the faster moving parts of the Earth system, the atmosphere, and the oceans. When it comes to the sedimentary system, the deep ocean base, we’re still struggling to see any impact where we can say with confidence is going to be a recognisable layer in the future that we can point to. That’s one test for defining new epochs; okay, after a few million years, will we be able to see where that new layer is? Will it preserve itself in the rock record and is it synchronous everywhere on the globe?
Q. Which is probably not always the case?
A. No, not always the case. You lose temporal resolution as you go back in time in the sediments, because everything gets compressed. From a human perspective it’s seemingly not synchronous at present because we can point to the bomb testing and plastics etc being different times, when it all gets compressed into the sedimentary record and if we were able to revisit Earth in a hundred million years from now, would it then appear to be synchronous? So, we should be conscious of our very limited perspective on time as humans as well.
“The Pleistocene is the period of Earth’s history when there were lots of ice ages, the last couple of million years. Typically, people would say Pleistocene/ice age to mean the same thing, but actually there were several ice ages in that time”
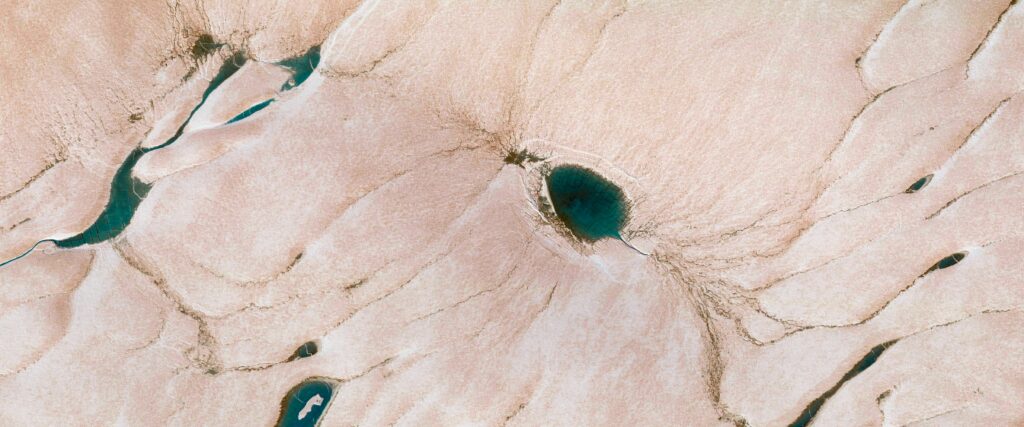
Q. You work on the Pleistocene epoch, tell us about that, is that the ice age?
A. Well yes, there were many ice ages. The Pleistocene is the period of Earth’s history when there were lots of ice ages, the last couple of million years. Typically, people would say Pleistocene/ice age to mean the same thing, but actually there were several ice ages in that time, and the one we have most evidence for of course is the last one. Often what happens is that you get multiple ice ages in the same place. On the continents, the last event tends to obliterate the evidence from the one before and so on, unless you look in a cave or somewhere out of harm’s way that could have been protected from the new ice. In the deep oceans, where sedimentary layers can build up slowly over time, we have evidence for multiple glacial/interglacial cycles going back through the Pleistocene. The same is true for the thick ice sheets that occur in places like Greenland and Antarctica, where the oxygen isotope ratios in the ice record changing temperatures in a cyclical way, pointing to repeated glacial/interglacial cycles during the Pleistocene.
“The appeal of Pleistocene is you can potentially get much more detailed time resolution that helps us to understand how the Earth’s climate system works, because you’re looking at a younger time interval”
Q. Why are you interested in this time period?
A. Partly my interest came from using the uranium-thorium isotope dating method, where we could date materials or rocks or sediments that were less than about 500 thousand years old. That encompasses a lot of the better-preserved Pleistocene materials, so we had this dating method that could be used. It was also at a time in my career, in the early 1990s, when climate change was starting to become more of a topic in Earth Sciences, and a lot of the older existing climate records from the continents and also from the oceans were not very well dated. Often, it was the case that scientists had developed some kind of proxy measurements for climate change, for instance it could be a change in the distribution of fossils like foraminifera, in a marine sediment core, but the chronology wasn’t well established.
Many palaeoclimatologists were simply tuning the chronologies of their marine sediment cores to the presumed astronomical forcing. We know that predictable changes in the Earth’s orbit around the sun causes natural climate variability on 100,000 year timescales and many scientists were superimposing that presumed chronology on the wiggles they could see in their various proxy data measurements from the sediments. It was a bit unsatisfactory because it risks a circular argument and doesn’t allow one to test the implications of real leads and lags between the orbital forcing and the response of the Earth’s climate system.
So, this uranium-thorium chronology approach provided a different, independent way of dating these Pleistocene climate change events. We started by dating fossil corals, because coral terraces are sensitive to relative sea level changes that in turn are linked to glacial/interglacial cycles. Sea level falls in a glacial, because more water is locked up as ice near the Earth’s poles, the corals get exposed and stop growing, so you can date times of rapid sea level change by dating the coral terraces. This helped to tie down the chronology for the last interglacial period. Then we started working on cave deposits, they are quite numerous on the continents and only grow in the presence of liquid water, in other words in ice-free interglacial conditions.
I suppose the appeal of Pleistocene is you can potentially get much more detailed time resolution that helps us to understand how the Earth’s climate system works, because you’re looking at a younger time interval, so all the sedimentary rocks haven’t really been squashed and compacted into thin layers or otherwise obliterated by later events.
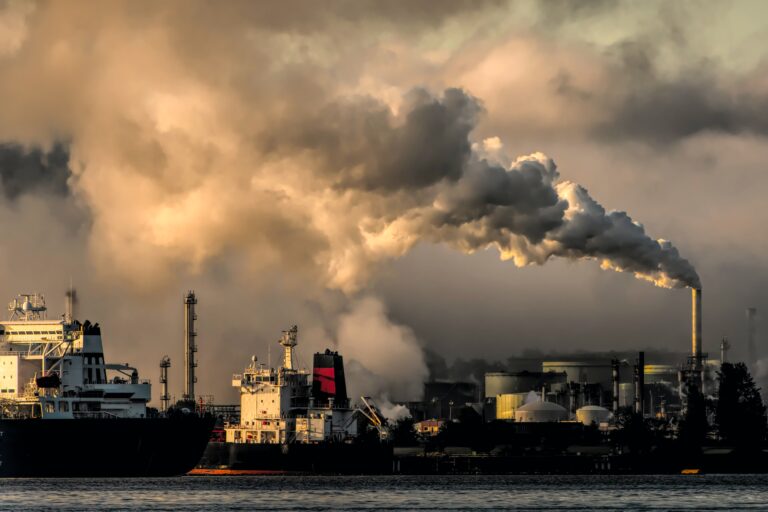
Q. So you can see when there were big changes, which can help to understand patterns?
A. Yes, the Pleistocene perspective helps us to understand what would happen in a baseline climate system if there were no anthropogenic forcing. What would we expect to be happening now in the absence of human emissions of greenhouse gases to the atmosphere? From the Pleistocene perspective, we’d expect the Earth to be cooling now; we should be slowly going back into an ice age. It’s interesting, if you go back and look at the scientific literature that was published in the 60s or so, the big concern then was that we’re going to soon have another ice age. And in a way it makes the current global warming that is now evident even worse, because the system should be heading towards a cooler state if humans hadn’t messed it up by injecting vast quantities of greenhouse gases into the atmosphere.
“The ice-cores are great because you can see the major climate cycles in them over the past few hundred thousand years. They are regarded as the best climate records, because they are reasonably well dated by counting visible annual layers. Each year is supposed to have a layer in the ice.”
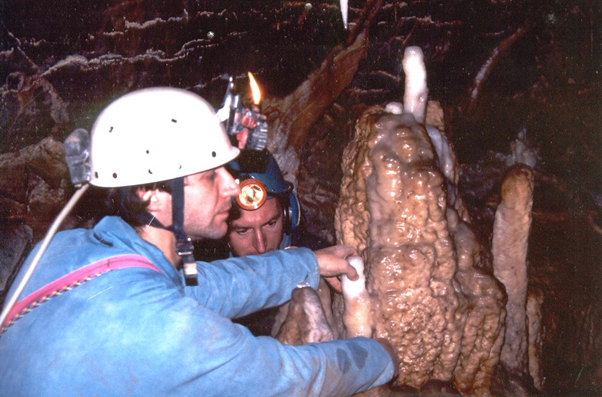
Q. This kind of work on past climates is called paleoclimatology – what kind of records would you work with in general?
A. Stalagmites would be my main source of information about past climate; I have done a lot of work on them over the past 30 years. Stalagmites grow in caves where calcium-saturated water is dripping in from the ceiling. First of all, if you’ve got drip-water, it’s already a first-order climate signal, because if the climate was cold and dry the soils and shallow rocks would remain frozen for most of the year and water would stop dripping into caves. When that happens, you get a pause or hiatus in the deposition of a stalagmite, and when the climate gets warm and wet again the stalagmite can start growing again. So, for a first-order climate signal, just dating those pulses of growth precisely by uranium-thorium isotopic dating of the calcite layers above and below hiatuses in stalagmites can tell you a lot about the timing of cold dry glacial times versus warm-wet interglacial times; how long did the cold-dry events last for, were they regionally important and so on.
When we correlated records of stalagmite deposition in Ireland with the data from the ice cores in Greenland the results were very interesting. As you know, ice cores extending for several kilometres in depth were drilled by European and American consortiums back in the 1990s, and over the years they have been analysed for all kinds of isotope and chemical tracers by many researchers. The ice-cores are great because you can see the major climate cycles in them over the past few hundred thousand years. They are regarded as the best climate records, because they are reasonably well dated by counting visible annual layers. Each year is supposed to have a layer in the ice.
When we compared our data for stalagmites from Irish caves can were able to see all of the Greenland events in Ireland. When it’s warmer in Greenland we get stalagmites being deposited in Ireland, even for short century-scale intervals of warmth that are now known to occur during glacial periods. In essence we’re tracking the extent to which climate signals are regionally developed as opposed to just local signals in Greenland. What’s more, the chronology from the Irish speleothems proved to be more accurate than that published for the Greenland ice cores as you go back further time, indicating that occasionally the Greenland ice core is missing annual layers.
“CO2 makes carbonic acid when it interacts with rainfall, the carbonic acid gets neutralised when it’s exposed to rocks during weathering processes, so you’ve essentially got a sink for CO2 in the ground.”
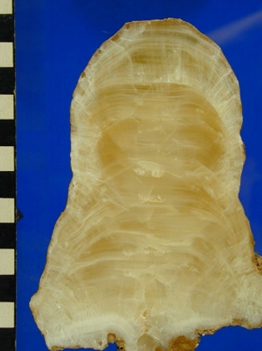
Image: stalagmite (cave deposit that grows from the floor of a cave). It is made of the mineral calcite and you can see layers that represent time – oldest in the middle at the bottom, all the way to youngest at the top/outer layers. The scale bar on the side is in centimetres, so this one is about 13cms tall and grew over a time interval of about 3,000 years in the late Holocene. We can use the changing chemistry of the layers to work out past environments above the cave (climate and soil-related changes).
Q. Another thing you’ve been working on recently is mineral weathering – can you explain what it is and its potential to mitigate climate challenges?
A. Mineral weathering happens naturally all the time. It’s one of the processes that regulates atmospheric CO2. Let’s say, for example back 100 million years ago, there was a big volcanic eruption and a lot of CO2 gets injected into the atmosphere, then the climate gets warmer, and we’ve had several examples of this kind of thing in the geological past. You get warming after these big volcanic eruptions. The question is how does the climate system recover? It recovers by weathering, by removing CO2 from the air. That’s because the CO2 makes carbonic acid when it interacts with rainfall, the carbonic acid gets neutralised when it’s exposed to rocks during weathering processes, so you’ve essentially got a sink for CO2 in the ground. During the weathering process, the CO2 in the air gets converted to dissolved bicarbonate in the water, in the streams, rivers and that bicarbonate basically runs out to the oceans where it remains dissolved for about 100,000 years on average.
There’s a transfer of carbon from the mantle, via the volcanoes, via the atmosphere via weathering, into the oceans. The key point here is that weathering is a negative feedback mechanism that stabilised Earth’s climate because weathering rates are faster when the climate warms, for example by volcanic injections of CO2 into the atmosphere. More CO2 leads to warmer conditions, leads in turn to more weathering which then pulls CO2 out of the air and returns the system to the original state – it’s a classic negative feedback or damping mechanism that prevents runaway warming.Wha
t we are trying to do is accelerate that natural process by effectively grinding up some rocks and other materials and putting them on soils as soil additives. The idea of putting them on soils is that soils are areas of very high CO2 because the plants are pushing out CO2 through their roots, through respiration, so you have a lot of carbonic acid there that can do the weathering. Basically, you’re giving the carbonic acid a chance to weather something that’s easy to weather like a small ground-up mineral grain, and it should draw down CO2? That’s the plan. There’s a lot of work going on all around the world on this. Potentially it could be a way to compensate for some or all the CO2 that humans have put into the air by burning fossil fuels.
Q. And these rock materials added to the ground could come from buildings?
A. Yes, we’re looking at the possibility of using crushed returned concrete to capture CO2 when it is added to soils and slowly breaks down by weathering. We already know that concrete in buildings takes up some CO2, partially offsetting the vast amounts of CO2 that are released when its main ingredient, cement is manufactured. It’s a small amount relative to what humans have released to the atmosphere, but it all helps I suppose. It’s not an alternative to decarbonisation of course, but given that we also have to reduce the amount of carbon already in the atmosphere, it could help.
Q. What kinds of issue do you think is the biggest challenge for the future in relation to climate and environment?
A. I think we have to begin thinking about the environment in a more holistic way. I guess the main challenge is how to keep economic development going but not degrade the environment any more than we have already. Policies need to be better aligned. In Ireland there seems to be a mismatch between food production policy and environmental policy for example. Nitrate levels in our surface and groundwaters are a huge challenge, as are methane emissions from agriculture. I think we must implement the right policies and to align our efforts better than we currently do.
Q. There is a fear that we will run out of certain things, like phosphorous for instance?
A. There’s certainly concern that phosphorous is running out. I don’t know if it’s quite as bad as some people claim; there’s still large phosphate reserves globally that haven’t been mined but we must get smarter about recovering phosphorus and other elements from waste streams. But I guess that brings us on to another point about mining. You know how mining is often seen as very bad environmentally, but we must do it because we need phosphorus and we need metals for decarbonisation, lithium and cobalt for example, if we are going to have electric cars. We must extract metals to decarbonise our energy system on a global basis. Even building a better electricity grid that can utilise distributed renewable energy sources such as rooftop solar needs new metals.
“[In Ireland] we have rocks ranging from Paleoproterozoic metamorphic rocks in Inishtrahull and North-West Mayo to widespread recent Quaternary sediments. Not all geological epochs are represented on the island of course, but if you’re teaching field geology here it’s brilliant.”
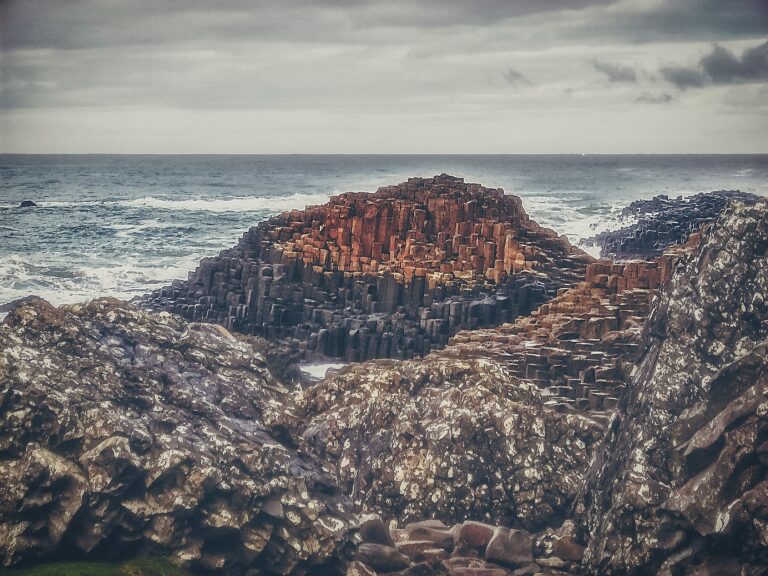
Q. Finally, looking at Ireland as a geologist, are there any unusual features about our island?
A. I guess the stunning thing about Ireland is its geological diversity. Given its small footprint as an island, we have an amazing range of geological time periods represented and different rock types and we shouldn’t forget that Ireland also has a vast and lightly explored offshore area that is several times larger than the island. We have rocks ranging from Paleoproterozoic metamorphic rocks in Inishtrahull and North-West Mayo to widespread recent Quaternary sediments. Not all geological epochs are represented on the island of course, but if you’re teaching field geology here it’s brilliant. Just on our doorstep, right along the DART line, you’ve everything from Cambrian metamorphic rocks through granites and contact metamorphic rocks to sedimentary rocks such as limestone. And you’ve got all the Quaternary deposits… the geological diversity in such a small area is amazing. If you landed randomly somewhere else in the world, I’d bet you’d be unlikely to find such geological diversity within a few kilometers of the capital city. We’re pretty lucky!